UNDERSTAND PHYSICS :TOPIC ELECTROMAGNETISM
Magnetic Fields due to a Current-carrying Conductor
How Electric Current Produce a Magnetic Field
Explain how electric current produces a magnetic field
Electromagnetism
is the effect produced by the interaction of an electric current with a
magnetic field. The interaction can result in a force on the conductor
carrying the current.
If,
on the other hand, a force is applied to a conductor (with no current)
in a magnetic field the resulting movement can result in a current being
noticed in the conductor.
When
the switch is closed an electric current flows through the conductor.
The electric current generates magnetic field around the conductor. This
will cause a deflection on the compass needle. The magnetic field
around a current-carrying conductor can be shown by means of magnetic
field lines.
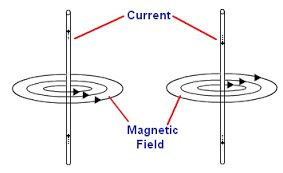
The Pattern of the Magnetic Field Lines around a Straight Conductor
Identify the pattern of the magnetic field lines around a straight conductor
The
magnetic field pattern is usually given in a plain view. In the plain
view, the conductor is represented by a circle. A dot in circle shows
that the current is coming out of the plane. A cross the circle shows
that the current is moving into the plane.
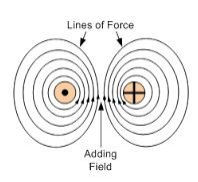
The
strength of the magnetic field on the magnitude of the electric
current. The higher the current, the stronger the magnetic field, and
therefore the greater the deflection. The strength of the magnetic field
decreases as you move further from the conductor. There will be less
deflection as the compass is drawn from the current-carrying conductor.
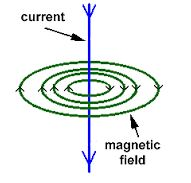
The Direction of Magnetic Field around a Current-Carrying Conductor
Determine the direction of magnetic field around a current-carrying conductor
The direction of the field is determined by applying two rules, these are:
- Right-hand Grip Rule
- Maxwell’s cork screw rule
Right-hand Grip Rule
The
Right-hand Grip Rule can be applied to a straight conductor or a
solenoid-carrying an electric current. For a straight conductor, the
Right-hand Grip Rule can be stated as:
“Imagine
the wire carrying the current is gripped by the right hand with the
thumb pointing in the direction of the conventional current (from
positive to negative), the fingers will curl around the wire pointing in
the direction of the magnetic field.”
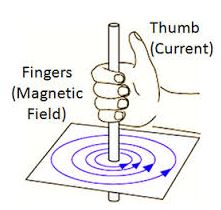
For a solenoid, the Right-hand Grip Rule states that:
“When
you wrap your right hand around a solenoid with your fingers pointing
in the direction of convectional current, your thumb point in the
direction of the magnetic North pole.”
A solenoid is a long coil containing a large number of close turns of illustrated copper wire.
Maxwell’s –Right –hand screw rule states that:
“If
a right-hand screw advances in the direction of the current, then the
direction of rotation of the screw represents the direction of the
magnetic field due to the current.”
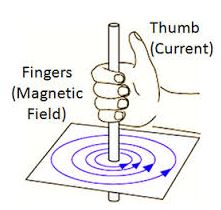
The Presence and Direction of a Force on a Current carrying Conductor in a Magnetic Field
Determine the presence and direction of a force on a current carrying Conductor in a magnetic field
The
direction of the force on a current-carrying conductor in a magnetic
field can be determined using Fleming’s Left –Hand Rule.
Fleming’s Left –Hand Rule states that:
“If
you hold the index finger, the middle finger and the thumb of your left
hand mutually perpendicular to each other so that the index finger
points in the direction of the magnetic field and the middle finger
points in the direction of current in the conductor, then the thumb will
point in the direction of the force acting on the conductor.”
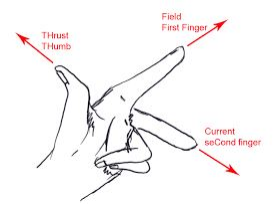
The Direction of Force due to two Current carrying Conductors when the Current Flowing in the Same or Opposite Direction
Determine
the direction of force due to two current '82air-carrying conductors
when the current flowing in the same or opposite direction
If
two current-carrying conductors are placed side by side close to one
another, the currents in the conductors will interact with the magnetic
fields produced by the two conductors. A force may result depending on
the direction of the two currents.
When
the currents are flowing in opposite directions, the conductors repel
one another. When the currents are flowing in the same direction, the
conductors attract one another, the conductors attract each other.
When
the currents flow in the same direction, the magnetic field between the
conductors cancel out, thus reducing the net field. However, on the
outside, the magnetic fields add up, thus increasing the net field.
Therefore, the magnetic field is weaker between the conductors that on
the outside. The resultant force pushes the conductor towards each
other.
When
the currents are in the opposite directions, the fields between the
conductors add up, while they cancel out on the outside. The field
between them is stronger than on the outside. The resultant force is
toward the outside of each conductor, hence repulsion.
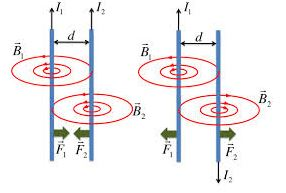
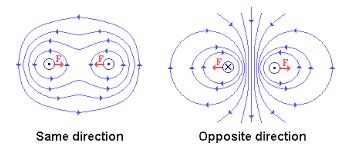
ElectroMagnetic Induction
The Concept of Electromagnetic Induction
Explain the concept of electromagnetic induction
Electromagnetic
Induction is the production of e. m. f whenever there is a change in
the magnetic flux linking a conductor”. The e. m. f produced is called
induced e. m. f and the resulting current induced current.
The Laws of ElectroMagnetic Induction
State the laws of electroMagnetic induction
Lenz’s Law
This
explains the direction of the induced e. m. f and it states that: “The
direction of the induced e. m. f is such that the resulting induced
current flows in such a direction that it opposes the change that causes
it.”
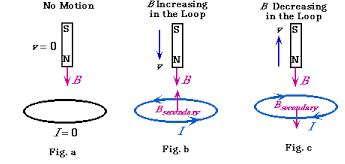
Faraday’s Law.
It
relates the magnitude of induced e. m. f and the rate of change of the
magnetic flux linking the conductor. The magnitude of the induced e. m. f
depends on:
- the strength of the magnetic field.
- the rate of change of the magnetic flux(speed of motion)
- the area of the conductor that is in the magnetic field.
Faraday’s
law states that: “The e. m. f induced in the conductor in a magnetic
field is proportional to the rate of change of magnetic flux linking the
conductor.”
The Concepts of Self and Mutual Induction
Explain the concepts of self and mutual induction
When the current flowing through a conductor varies it creates a varying magnetic field that cuts across the conductor itself.
This
results to self-induced e. m .f in the conductor that is opposite in
direction to the original e. m. f. This voltage, usually referred to as
back e. m .f, tends to limit or reverse the original current.
If
the original current is increasing, then the induced current subtracts
from it and then measured current is smaller than it would be if no
self-induced magnetic field was produced in the conductor. If original
current is decreasing, then the original current adds to it and the
measured current is greater than it would be if self-induced magnetic
field was produced in the conductor. This process is called
self-induction since the changing current creates a back e. m. f in
itself.
Consider
a coil of wire wrapped around a cardboard tube. When the coil is
connected to a battery a current flows in the coil producing a magnetic
field as shown below.
If
the current in the coil begins to increase, the magnetic flux
increases. This induces an e. m. f that opposes the battery resulting in
a back current that impedes the increase in current. If the current in
the coil decreases, the magnetic flux decreases. This induces an e. m .f
that adds to the battery resulting in an induced current that impedes
the decrease in current.
If
we place two coils near each other, a varying current in one coil will
induce a current in the other. This is called mutual induction. The coil
with a changing current is referred to as the primary coil while that
in which a current is induced is the secondary coil.
The e.m.f induced in the secondary coil is proportional to the rate of change of the current in the primary coil.
The Mode of Action of Induction Coil
Describe the mode of action of induction coil
Is
an electrical device consisting of two coils, the primary coil and the
secondary coil, wound one over the other on an iron core.
It
is used to produce high-voltage alternating current from low-voltage
direct current. The primary coil is made up of tens or hundreds of turns
of coarse wire while the secondary coil consists of thousands of turns
of fine wire. The secondary coil is wound on top of the primary coil.
Mode of action
An
induction coil produces high voltage in its secondary coil by
electromagnetic induction. The direct current in the primary is switched
on and off by a make-and-break mechanism. This produces change in
current and magnetic field which is necessary for electromagnetic
induction to occur in the secondary coil.
When
the current in the primary coil is switched on, the induced magnetism
in the iron core attracts the soft-iron armature.The moving iron
armature opens a gap between the two contacts which breaks the primary
coil circuit. This switches off the current. As the induced magnetism
fades away, the armature springs back, closes the contacts and completes
the circuit again. This allows the current to flow in the primary coil
again. This cycle of events is repeated automatically.
The
induced a.m. is very large, usually in the order of hundreds of
kilovolts (kV).Such a high voltage is achieved because of two things:
- The secondary coil has a large number of turns compared to the primary coil.
- The rapid change in the primary current when it is switched on and off causes a rapid in the magnetic field through the secondary coil.
Applications of the induction coil
- It is used in the ignition system of internal combustion engines.
- It is used to trigger the flash tubes used in cameras and strobe lights.
- It is also used in wireless telegraphy.
The Mode of Action of a.c and d.c Generator
Describe the mode of action of a.c and d.c generator
A
generators is the device which produces electricity on the basis of
electromagnetic induction by the continuous motion of either a coil or a
magnet.
A.C Generator or alternator
An
arc generator utilizes Faraday’s law of induction, spinning a coil at a
constant rate in a magnetic field to induce an oscillating a.m.
The
arc generator consists of an armature made up of several turns of
insulated wire wound on a soft-iron core. The armature revolves freely
on an axis between the poles of a powerful magnet, which provides a
strong magnetic field. Two slip rings are connected to the ends of the
armature and two carbon brushes rest on the slip rings.
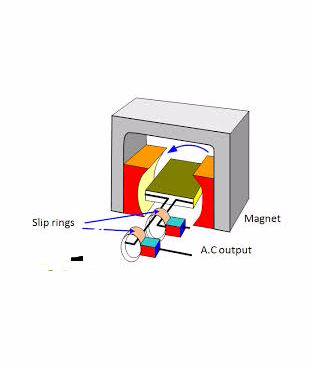
When
the coil is vertical, no cutting of the magnetic lines of force takes
place although the number of lines linking the coil is maximum. The rate
of change magnetic flux is zero and as a result, no a.m. is induced in
the coil.
When
the armature is parallel to the magnetic field, the rate of change of
magnetic flux is maximum and the motion of the coil is perpendicular to
the magnetic field, hence an a.m. is induced along the sides of the
coil.
After
a 180° turn, starting from the vertical position, the sides of the loop
interchange and the current in the loop is reversed. This means that
the a.m. is positive for one half of the cycle and negative for the
half. The maximum induced a.m. is at 90° rotation from the vertical
position and the minimum is at 270° rotation. If there is an external
circuit, the current through it would also have a maximum value at 90°
and minimum at 270°.
This
kind of current is called an alternating current and the corresponding
a.m. is the alternating e.m.f.The number of cycles produced per second
is called the frequency of the arc. The arc obtained is led to an external circuit through the slip rings and the carbon brushes.
D.C generator
It
is made by replacing the slip rings in the arc generator with a
commentator. Each half of the commentator ring is called a commentator
segment and is insulated from the other half. Each end of the rotating
loop of the wires connected to a commentator segment. Two carbon brushes
connected to the outside circuit rest against the rotating commentator.
In
the deck generator, the commentator rotates with the loop of wire, just
as the slip rings do with the rotor of an arc generator.
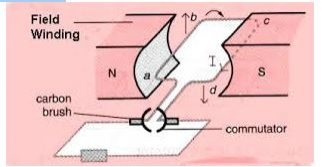
When
the loop is rotated in the magnetic field, the induced e.m.f is still
in alternating form. However after rotation of 180° instead of the
current reversing, the connections to the external circuit are reversed
so that the current direction in the external circuit remains the same.
The output of a d.c generator is shown below.
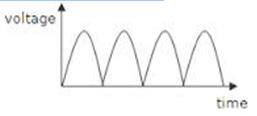
Note:The lower half of the cycle is not cut off but is reversed.
Simple Step-up and Step-down Transformer
Construct a simple step-up and step-down transformer
A
transformer is the device that uses mutual induction between two coils
to convert an a.c across one coil to a larger or smaller a.c across the
other coil.
A
transformer is made up of two coils, each with a different number of
loops linked by an iron core so that the magnetic flux from one passes
through the other. When the flux generated by one coil changes the flux
passing through the other will change, inducing a voltage in the second
coil.
The coil that provides the flux that is the coil connected to the a.c power source is known as the primary coil while the coil in which the voltage is induced is known as the secondary coil.
When
the number of turns in the primary coil (N) is lower than the number in
the secondary coil (N),the secondary voltage will be lower than the
secondary voltage. This is called the step-down transformer. The opposite of this is called the step-up transformer.
No comments